80 ps FHWM Instrument Response with ID230 InGaAs
SPAD and SPC‑150 TCSPC Module
Wolfgang Becker, Holger Netz, Becker & Hickl GmbH
Michael Desert, ID Quantique
The new ID
Quantique ID230 InGaAs SPAD delivers an instrument response width of 80 ps
FWHM with the bh SPC-150 TCSPC module. This is the fastest TCSPC response
reported for InGaAs SPADs so far. Compared to its predecessor the new InGaAs
SPAD also has a much lower dark count rate. The detector we tested had less
than 300 dark counts when operated with a dead time of 40 µs. Optical
signals as weak as 800 photons per second count rate could thus be
detected at high signal-to-background ratio. Results are presented in this
application note.
TCSPC With InGaAs SPADs
Single-photon avalanche diodes (SPADs) based
on InGaAs material achieve high detection efficiency in the wavelength range
from 900 nm to 1700 nm. A problem of early InGaAs SPADs was the high
dark count rate, and the strong afterpulsing. To avoid instability, the devices
could only be operated in a gated mode: The reverse voltage is increased above
the breakdown level for a period of 10 to a few 100 ns, and then decreased
below the critical level for several microseconds. Gated operation may be a
solution for general photon counting in combination with low-repetition rate
light sources. It is, however, not acceptable in combination with TCSPC: TCSPC
works best at high repetition rate of the light signals, and requires the
detector to be active continuously. With early InGaAs SPADs pseudo-continuous
operation at low dark count rate could be obtained by gating the detector with
an asynchronous HF signal. However, this reduces the effective detection
efficiency by several orders of magnitude. Efficiencies and dark count rates
reported for this kind of operation should therefore considered with
reservation.
The first InGaAs SPAD for true continuous
operation was the id220 detector of ID Quantique [1]. The ID220 delivers an IRF
width of about 220 ps with the bh TCSPC modules. The dark count rate is on
the order of a few 1000 to a few 10,000 counts per second, depending on the
detector parameters selected. Results for fluorescence-decay recording and
confocal FLIM have been presented in [3] and [6]. In 2015 ID Quantique
released a new InGaAs SPAD, the ID230, with substantially improved performance
[2]. The detector is shown in Fig. 1. We have tested the ID230 in combination
with a bh SPC‑150 TCSPC module and a bh BDS‑SM-1064 nm
picosecond diode laser. Results are presented in this application note.
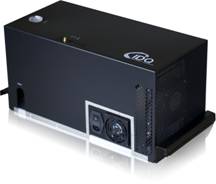
Fig. 1: ID230
InGaAs SPAD detector
Results
For characterising the id230 in combination
with the bh TCSPC modules we used the setup shown in Fig. 2. A BDS‑SM
picosecond diode laser [7] generates
a 50 MHz train of picosecond light pulses at a wavelength of 1064 nm.
The BDS-SM laser has a single-mode fibre output. The light from the laser fibre
was injected into the multi-mode input fibre of the ID230 through a package of ND
filters. The fibres were aligned face-to-face without any focusing optics in
between. The input fibre of the detector was thus illuminated at low numerical
aperture. This is important to minimise pulse dispersion in the fibre. One
meter of multi-mode fibre can introduce almost 100 ps of pulse dispersion
when used at maximum NA [5]. This
is on the same order as the expected IRF width of the id230 detector. An
optimum IRF width can thus only be achieved by keeping the NA at the input of
the detector fibre low.
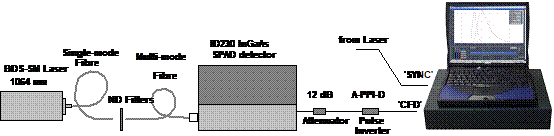
Fig. 2: TCSPC
setup for characterising the id230 detector
The TCSPC system was a standard bh
Simple-Tau 150, containing an SPC‑150 TCSPC module [6]. The detector output pulses were adapted
to the input voltage range of the SPC module by an attenuator and an A‑PPI-D
pulse inverter. The synchronisation signal for the TCSPC module was obtained
directly from the Sync output of the BDS-SM laser. A typical laser pulse shape
recorded with this setup is shown in Fig. 3.
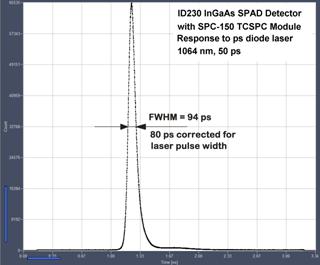
Fig. 3: Laser
pulse recorded with the Id230 SPAD. BDS-SM-1064nm ps diode laser, SPC-150 TCSPC
module. Detection efficiency 25%, dead time 5 µs. Laser pulse width is 50 ps
to 60 ps.
The width of the recorded photon
distribution is 94 ps. These 94 ps necessarily contain a contribution
from the laser pulses. The width of laser pulses is not exactly known since we
had no fast reference detector for a wavelength of 1064 nm. However,
BDS-SM lasers of sub-1000 nm wavelength have pulse widths in the range of
50 to 60 ps. Assuming the same width for the pulses at 1064 nm
the real IRF width of the detector-TCSPC combination can be expected in the
range of 72 ps to 80 ps. To our knowledge, this is the fastest TCSPC
response reported for an InGaAs SPAD yet.
Operating Conditions of the Detector
The ID230 detector comes with software that
allows the user to adapt the detector parameters to the conditions of the
experiment. Both the detection efficiency and the dead time (quenching time
after detecting a photon) can be modified. The best TCSPC results were obtained
with the detection efficiency set to the maximum of 25%. Reducing the detection
efficiency results in a broader IRF and is therefore not recommended.
Unlike the detection efficiency parameter,
the dead time selection in the ID230 software is important to optimise the
TCSPC performance for different experiments. Shorter dead time results in
higher saturated count rate and less counting loss but also in higher background
and stronger afterpulsing. For typical applications with count rates in the 10 kHz
to 100 kHz range a dead time of 5 µs was found to be the best compromise. For
low-count-rate applications longer dead times should be used. At count rates in
the range of a few kHz or below an increase in dead time does not cause noticeable
loss of photons. It does, however, result in a dramatic decrease of the
counting background. An increased dead time thus increases the dynamic range of
the recording.
An example is shown in Fig. 4. A signal
that delivered only 800 counts per second was recorded with a dead time of
40 µs. At 800 counts per second, the loss of photons in the 40 µs following
a previously recorded photon is negligible. However, the increased dead time
reduces the dark count rate from several 1000 down to less than 300 counts
per second. The dynamic range therefore increases, and the signal is recorded
at high signal-to-background ratio. The result shows that the ID230 can be used
down to extremely low light intensities.

Fig. 4: Laser pulse recorded at a count rate of 800 photons per
second. ID230 dead time 40 µs, acquisition time 600 seconds. The long
dead time decreases the counting background and thus results in a high
signal-to-background ratio.
Summary
With its 80 ps IRF width and 25%
detection efficiency the combination of the ID230 InGaAs SPAD and the bh SPC‑150
TCSPC module records optical signals in the IR at unprecedented time resolution
and sensitivity. Faster detection at IR wavelengths has only been obtained with
superconducting detectors [4].
References
1.
ID Quantique, ID220-FR Cost-effective module for
asynchronous single-photon counting at telecom wavelengths. See idquantique.com
2. ID Quantique, ID230 data sheet, see www.idquantique.com
3. Becker & Hickl GmbH, TCSPC at Wavelengths from 900 nm to 1700
nm. Application note, see www.becker-hickl.com
4. Becker & Hickl GmbH, World Record in TCSPC Time Resolution:
Combination of bh SPC-150NX with SCONTEL NbN Detector yields 17.8 ps FWHM.
Application note, see www.becker-hickl.com
5. W. Becker, Advanced time-correlated single-photon counting techniques. Springer,
Berlin, Heidelberg, New York, 2005
6.
W. Becker, The bh TCSPC handbook. 6th edition,
Becker & Hickl GmbH (2015), available on www.becker-hickl.com
7. BDS-SM picosecond diode lasers. Extended datasheet. Available on
www.becker-hickl.com
Contact:
Wolfgang Becker Michael
Desert
Becker & Hickl GmbH ID
Quantique
becker @becker-hickl.com michael.desert@idquantique.com