Sub-20ps IRF Width from Hybrid Detectors and MCP-PMTs
Wolfgang Becker,
Vladislav Shcheslavskiy, Becker & Hickl GmbH, Berlin, Germany
Abstract: Hybrid detectors and multichannel-plate
(MCP) PMTs achieve a timing resolution (IRF width) of less than 20 ps FWHM
when operated with the new bh SPC-150NX TCSPC modules. As a test light source,
we used a Toptica FemtoFErb laser with a pulse width of 100 fs. The laser
beam was directed through a package of ND filters to the photocathodes of a bh
HPM-100-06 and a HPM-100-07 detector (based on Hamamatsu R10467-06 and ‑07
tubes) and a Hamamatsu R3809U-50 MCP PMT. In all cases, an IRF width around
20 ps FWHM and below was obtained. This is considerably shorter than previously
reported for these detectors. We attribute the improvement to the superior
bandwidth of the SPC‑150N discriminators and the extremely low timing
jitter of the timing electronics of the SPC-150NX modules.
The Instrument Response Function
The time resolution of a TCSPC system is
characterised by the width of the Instrument Response Function, or IRF. The
IRF contains the width of the excitation pulses, the timing jitter in the
detector, the timing jitter and amplitude-noise-induced jitter in the
discriminators for the detector and reference pulses, and timing jitter in the
time-measurement electronics [1, 2]. The width of the IRF is usually given as
full width at half maximum, or FWHM. Occasionally one can find the IRF
width (incorrectly) specified by an RMS (root mean-square) value. The RMS
value is the timing variance of the photons. The timing variance is about 2.5
times smaller than the real FWHM of the IRF.
The bh SPC-150N and SPC-150NX TCSPC modules
have ultra-high bandwidth (5 GHz) discriminators, and internal timing
electronics with less than 6.5 ps and 3.5 ps FWHM timing jitter.
There were earlier indications that these modules deliver exceptionally short
IRFs with fast detectors: The IRF of a superconducting NbN detector was determined
to be 17.8 ps [3, 4], and HPM-100-06 and -07 hybrid detectors delivered surprisingly
short IRF widths of 38 ps with ps diode lasers [6]. We therefore
determined the IRF width for three fast detectors in combination with SPC-150NX
modules.
Measurement Setup
As a test light source we used a Toptica FemtoFErb
fibre laser with 100 fs pulse width. The laser wavelength was 785 nm,
the pulse repetition rate 100 MHz. The laser beam was sent directly
through a package of ND filters to the photocathode of the detector. The
synchronisation signal for the TCSPC module was taken from the synchronisation
output of the laser. The photon pulses of the PMH‑100-06 and ‑07
detectors were connected directly, the pulses from the R3809U-50 MCP via a HFAC-26
(26 dB, 1.8 GHz) preamplifier to the CFD input of the SPC-150NX module.
The operating voltage for the R3809U was generated by a bh HVM-3000 high-voltage
module. All detectors were operated via a bh DCC-100 detector controller card
which provided the operating voltages, gain control, and overload shutdown [2].
The principle of the test setup is shown in Fig. 1.

Fig. 1: Test setup for IRF measurement
Results
Hybrid Detectors
The IRFs measured for an HPM-100-06 and an HPM-100-07
detector are shown in Fig. 2. The FWHM of the IRFs are 18.9 ps and
19.4 ps, respectively. The curves were measured with a CFD threshold at
which all photon pulses were recorded. The CFD zero cross was adjusted for
minimum IRF width.

Fig. 2: Left: IRF of HPM-100-06 (bi-alkali cathode). Right: IRF of
HPM-100-07 (multi-alkali cathode). The full with at half maximum is
18.9 ps and 19.4 ps, respectively. SPC-150NX, 100 ps/div,
405 fs/channel
Both IRFs have a small afterpulse. The
shape and the amplitude of the afterpulse does not depend on the CFD threshold
or the CFD zero-cross level. It also does not depend on the distance between
the ND filter package and the photocathode. A reflection between the cathode
and the filters can thus be excluded. The afterpulse does, however, depend on
the location of the illuminated spot on the cathode, and on the angle of
illumination. We believe that it is caused by photons that pass the
photocathode and are reflected back by some internal structure inside the tube.
This assumption is supported by the fact that we do not see an afterpulse in
measurements with a fast 405-nm picosecond diode laser: The photocathode is
almost transparent at NIR wavelengths but nearly opaque in the blue region. Internal
reflections are thus less pronounced at 405 nm.
R3809U MCP-PMT
The IRF measured for an R3809U-50 MCP PMT
is shown in Fig. 3. The detector was operated at ‑3000 V, and with
an HFAC-26 (26dB, 1.8 GHz) preamplifier. As for all PMTs, the
single-photon pulses of MCP PMTs spread over a wide amplitude spectrum. Since
larger pulses deliver a better timing accuracy the IRF depends on the CFD
threshold. Fig. 3, left, shows the IRF for a CFD threshold that detects 93% of
the pulses (blue curve), and a threshold that detects only 43% (red curve). The
IRF width is 22.6 ps and 19.1 ps, respectively.
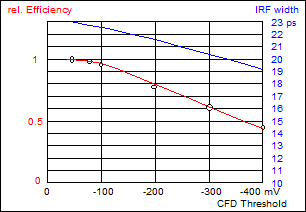
Fig. 3: Left: IRF for R3809U MCP PMT, operating voltage -3000 V. CFD
threshold -100mV (blue curve) and -400 mV (red curve). Right: Relative
efficiency and FWHM of IRF as a function of CFD threshold. 26dB preamplifier, operating
voltage ‑3000V, entire photocathode illuminated.
The dependence of the IRF width and the
detection efficiency on the CFD threshold is shown in Fig. 3, right. As can be
seen from Fig. 3, also the R3809U reaches an IRF width below 20 ps if a
moderate drop in detection efficiency is taken into account.
It should be noted that the IRFs shown in Fig.
3 were recorded at a detector operating voltage of ‑3000 V, and with
the entire photocathode illuminated. A count rate of several MHz can be
achieved under these conditions. Slightly shorter IRF width may be possible at
the maximum operating voltage of ‑3400 V, and with a smaller
illuminated area. However, the count rate is then limited by saturation of the
microchannels, and by exceeding the permitted output current of the detector [1,
2].
Fluorescence Decay Experiment
To make sure that the short IRF values are
not caused by artefacts like multiphoton triggering we recorded a fluorescence
decay curve of Indocyanin Green (ICG) in water with the HPM-100-07 detector.
ICG excites well at 785 nm, and has a fluorescence lifetime of
<200 ps in water. (The lifetime is longer in ethanol and when ICG is
bound to serum albumin) The result is shown in Fig. 4.

Fig. 4:
Fluorescence decay curve of ICG in water. The fluorescence lifetime is
175 ps.
A single-exponential fit with bh SPCImage
delivered a lifetime of 175 ps. A lifetime this short is on the order of
the IRF width of many lifetime spectrometers. With the HPM-100-07 and the SPC‑150NX
the decay function is so cleanly resolved that the shape of the recorded decay
curve is almost independent of the IRF.
Summary
With the bh SPC-150NX TCSPC module the
bialkali and multialkali hybrid detectors and the R3809U MCP PMTs enter the
exclusive club of sub-20-ps TCSPC detectors.
So far, similar IRF widths have only be
obtained with superconducting NbN detectors (see bh news 2017-02), and, in a
few cases, with MCP PMTs operated at maximum voltage and a CFD threshold that
detected only a small fraction of the photon pulses. However, NbN detectors
have extremely small active areas, and MCP PMTs operated at high CFD threshold
deliver poor efficiency and limited count rate. No such tradeoffs were made for
the tests described here: The light was spread over an area of about 5 mm2
for the hybrid detectors, and 50 mm2 for the MCP PMT. The MCP
PMT was operated at ‑3000 V (88% of the maximum), and at a CFD
threshold that suppressed no more than 50% of the photon pulses. The maximum
count rate under these conditions is several MHz. For the HPM detectors a CFD
threshold was used that did not suppress any photon pulses at all.
We believe that the detector / SPC
combinations described here deliver the best combination of time resolution,
detection area, and sensitivity currently available.
References
1. W. Becker, Advanced Time-Correlated Single-Photon Counting Techniques. Springer,
Berlin, Heidelberg, New York, 2005
2. W. Becker, The bh TCSPC Handbook. 9th edition, Becker & Hickl
GmbH (2021), available on www.becker-hickl.com
3. Becker & Hickl GmbH, World Record in TCSPC Time Resolution:
Combination of bh SPC-150NX with SCONTEL NbN Detector yields 17.8 ps FWHM.
Application note, available on www.becker-hickl.com
4. V. Shcheslavskiy, P. Morozov, A. Divochiy, Yu. Vakhtomin, K.
Smirnov, W. Becker, Ultrafast time measurements by time-correlated single
photon counting coupled with superconducting single photon detector, Rev. Sci.
Instrum. 053117 (2016)
5.
Becker & Hickl GmbH, SPC-150NX TCSPC/FLIM
module. Data sheet, avalable on www.becker-hickl.com
6. HPM-100-06/07 Ultra-High Speed Hybrid Detectors for TCSPC. Data
sheet, available on www.becker-hickl.com
Contact:
W. Becker, becker@becker-hickl.com