Implantable Fibre-Optical Fluorescence-Lifetime
Detection System for in-vivo Applications
Wolfgang Becker,
Ludwig Bergann, Becker & Hickl GmbH, Berlin, Germany
This application note describes a
fluorescence-lifetime detection system based on picosecond diode laser
excitation, a single-mode excitation fibre, one or several multi-mode detection
fibres, high-efficiency single-photon detectors, and a portable time-correlated
single photon counting (TCSPC) system. The excitation and detection system can
easily be connected and disconnected from the measurement object via
small-size, low-weight fibre connectors. The system records fluorescence decay
curves, phosphorescence decay curves, time-series of fluorescence decay curves,
intensity-traces, and fluorescence correlation data. By triggering the
acquisition with an external stimulation of the measurement object, dynamic
effects in the fluorescence lifetime and intensity down to millisecond range
can be recorded.
Picosecond Diode Lasers
Fluorescence excitation is performed by a
bh BDL-SMN or BDL-SMC picosecond diode laser. Available wavelengths are
405 nm, 445 nm, 473 nm, 488 nm, 515 nm, 640 nm,
685 nm, and 785 nm. The pulse width is on the order of 50 to
90 ps. The pulse repetition rate is switchable between 20 MHz,
50 MHz, and 80 MHz. Depending on the wavelength and the selected
repetition rate, the maximum available (average) laser power is from about 0.5
to several mW. The laser is shown in Fig. 1, left, typical pulse shapes in Fig.
1, right . The laser is operated from a simple +12V power supply; the driver
electronics is integrated in the laser head.
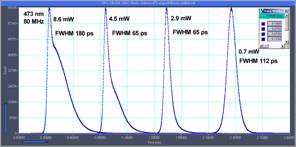
Fig. 1: Left:
BDL-SMN picosecond diode laser. Right: Pulse shapes for different laser power.
473 nm version, 80 MHz.
Fibre coupling system
Excitation and detection fibres are shown
in Fig. 2. Typical fibre core diameters are 3 µm for the excitation
fibres, and 25 µm for the detection fibres. The excitation fibres are
single-mode fibre fibres. This avoids motion artefacts by mode fluctuations
when the fibres are bent. The detection fibres are multi-mode to increase the
light-collection efficiency. Intensity changes by mode fluctuations do not
occur on the detection side because the fluorescence signal is diffusely
coupled and has a wide optical spectrum. Due to the small diameter the fibres
can be implanted into live animals, see [6, 7].
The excitation and detection fibres are
either separate (Fig. 2, left), or the fibres are mounted in a single
excitation/detection probe (Fig. 2, right).
Fig. 2:
Excitation and detection fibre (left), combined excitation/detection fibres
(right)
For in-vivo applications it is important that
the excitation and detection system can be connected and disconnected from the
measurement object. This is achieved by small-size
light-weight fibre connectors, Fig. 2, left, upper
left. Details of the connectors are shown in Fig. 3. The
total weight of the fibre connection is about 2 g.
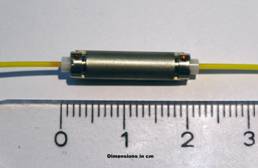
Fig. 3: Fibre
mini connectors for excitation and detection fibres. Unconnected (left) and
connected (right)
The principle of the fibre-based excitation
and detection system is shown in Fig. 4.

Fig. 4:
Principle of fibre excitation and detection system
Detectors
The fluorescence signals are detected by bh
HPM-100-40 hybrid detector modules [2]. The detectors combine excellent
detection efficiency up to about 700 nm, extremely clean and short
instrument-response function (IRF), and exceptionally low background signals.
For the near-infrared range up to 900 nm the HPM‑100-50 detectors
can be used. Emission filters can be inserted in the fibre adapters of the
detectors. To detect in two wavelength intervals simultaneously two HPM
detectors can by coupled to an optical beamsplitter assembly, see Fig. 5,
right.
Fig. 5: Left:
HPM-100 hybrid detector. Right: Two HPM-100 detectors coupled to a beamsplitter
assembly.
Multi-spectral detection is obtained by
using the bh PML‑SPEC multi-spectral TCSPC detector, see Fig. 6. This
detector is based on a PML‑16 16-channel PMT array and a polychromator.
It records simultaneously in 16 wavelength intervals [2, 3]. Since 2014, the PML-SPEC detector is
available with a GaAsP cathode [4]. This cathode has about 5 times the
efficiency of the bialkali cathodes of the older detector versions. The
PML-SPEC connects directly to the TCSPC system described below. The detector
uses bhs routing technique, therefore only one TCSPC module is needed to
record the data of the 16 channels. Compared to the HPM-100 detectors, the
PML-SPEC assembly has the advantage of spectral resolution. The disadvantage is
that some light is lost in the polychromator, and that the IFR is wider [2].
The use of the PML-SPEC is mainly recommended for autofluorescence applications
where spectrally resolved decay data provide essential information on the
measurement object.
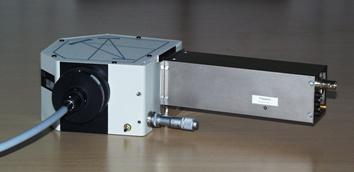
Fig. 6:
PML-SPEC spectrally resolved TCSPC detector
TCSPC System
The complete TCSPC electronics is contained
in an extension box connected to a laptop computer, see Fig. 7. The system is
modular. Additional experiment control modules or TCSPC modules can be added to
the system. Thus, depending on the type and the number of detectors used,
systems with one, two, three, or four parallel TCSPC channels are available.

Fig. 7: Simple-Tau
TCSPC system. Up to four TCSPC modules are contained in an extension box connected
to a laptop computer.
The TCSPC system uses bhs
multi-dimensional TCSPC technique [1, 2]. It is able to record fluorescence
decay curves, time-series of fluorescence decay curves, intensity-traces, and
fluorescence correlation data. Phosphorescence decay curves can be recorded
simultaneously with fluorescence data [2, 5]. By triggering the acquisition
with an external stimulation of the measurement object, dynamic effects in the
fluorescence lifetime and intensity down to microsecond range can be recorded. With
a multi-wavelength detector, such data can be recorded simultaneously in 16
wavelength intervals. Please see [2] for details.
References
1.
W. Becker, Advanced time-correlated single-photon counting techniques. Springer (2005)
2.
W. Becker, The bh TCSPC handbook. 6th edition. Becker
& Hickl GmbH (2014), www.becker-hickl.com. Becker, W., Su, B., Weisshart, K. &
Holub, O. (2011) FLIM and FCS Detection in Laser-Scanning Microscopes:
Increased Efficiency by GaAsP Hybrid Detectors. Micr. Res. Tech. 74, 804-811
3.
Becker & Hickl GmbH, 16 channel
detector head for time-correlated single photon counting, user handbook,
available on www.becker‑hickl.com, (2006)
4.
MW FLIM GaAsP detector. Data sheet,
www.becker-hickl.com
5.
Becker, W., Su, B., Bergmann, A., Weisshart, K.
& Holub, O. (2011) Simultaneous Fluorescence and Phosphorescence Lifetime Imaging.
Proc. SPIE 7903, 790320
6.
G. Cui, S.B.Jun, X. Jin, M.D. Pham, S.S. Vogel,
D.M. Lovinger, R.M. Costa, Concurrent activation of strial direct and indirect
pathways during action initiation. Nature (2013)
7.
G. Cui, S.B.Jun, X. Jin, G. Luo, M.D. Pham, D.M.
Lovinger, S.S. Vogel, R.M. Costa, Deep brain optical measurement of cell
type-specific neural activity in behaving mice. Nature Protocols, 9(6)
1213-1228 (2014)
Contact:
Wolfgang Becker, Becker &
Hickl GmbH, Berlin, Germany. Email:
becker@becker-hickl.com