
Principles
Table of Contents:
- Förster Resonance Energy Transfer (FRET) – Exploring Protein-Protein Interaction
- Understanding Protein Interactions with FLIM-FRET: How to Interpret Data and Visualize Results
- Double-Exponential FLIM-FRET Analysis – Accurate Distance Measurements
Förster Resonance Energy Transfer (FRET) – Exploring Protein-Protein Interaction
Förster resonance energy transfer (FRET) is an interaction of two molecules in which the emission band of one molecule overlaps the absorption band of the other. In this case the energy from the first molecule, the donor, can transfer into the second one, the acceptor. FRET can result in an extremely efficient quenching of the donor fluorescence and, consequently, in a considerable decrease of the donor lifetime. The energy transfer rate from the donor to the acceptor decreases with the sixth power of the distance. Therefore it is noticeable only at distances shorter than 10 nm . FRET is used as a tool to investigate protein-protein interaction. Different proteins are labelled with the donor and the acceptor, and FRET is used as an indicator of the binding between these proteins. FRET is the most frequent FLIM application.
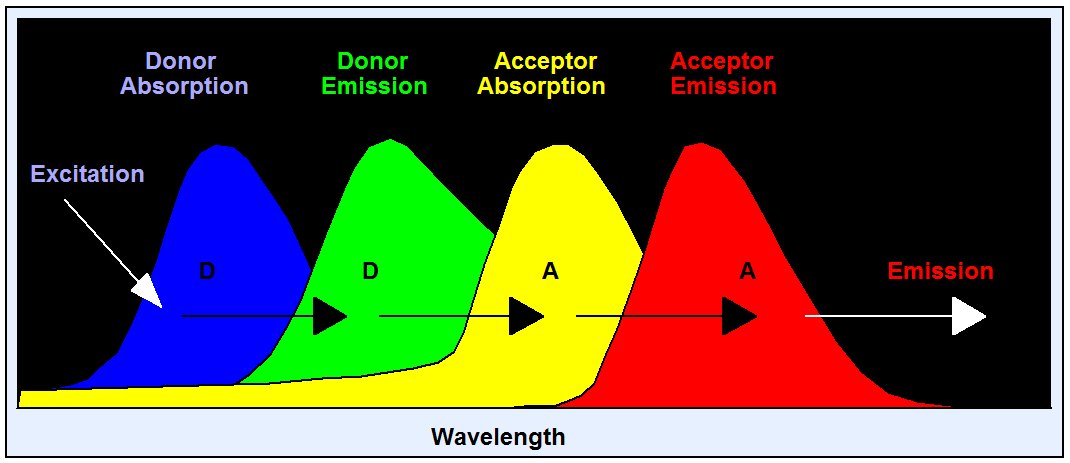
Because of its dependence on the distance FRET has become an important tool of cell biology. Different proteins are labelled with the donor and the acceptor; FRET is then used to verify whether the proteins are physically linked and to determine distances on the nm scale.
Understanding Protein Interactions with FLIM-FRET: How to Interpret Data and Visualize Results
The interpretation of FLIM-FRET data is simple: In the absence of FRET the lifetime of the donor is unchanged. When FRET is present the donor is loosing its excitation energy into the acceptor, and the lifetime decreases, see figure below.

The use of FLIM for FRET has the obvious benefit that the FRET intensity is obtained from a single lifetime image of the donor. When FRET occurs the fluorescence lifetime of the donor decreases, when FRET is absent it remains constant. All that is needed to detect protein interaction is a single lifetime image of the donor. Donor bleedthrough and directly excited acceptor fluorescence therefore have no influence of FLIM-FRET measurements. An example is shown in the figure below.

Double-Exponential FLIM-FRET Analysis – Accurate Distance Measurements
In all protein-interaction experiments, there is usually a mixture of interacting and non-interacting proteins. Both the fraction of interacting proteins and the distance between the proteins influence the net FRET efficiency derived from the intensities. It therefore cannot be told whether a variation in FRET efficiency is due to a variation in the distance or a variation in the fraction of interacting proteins.
TCSPC FLIM solves the problem of interacting and non-interacting donor by double-exponential lifetime analysis. The resulting donor decay functions can be approximated by a double exponential model, with a fast component from the interacting donor molecules and a slow lifetime component from the non-interacting donor molecules. There are several reasons why a donor does not interact. The protein may just not be linked to each other, an acceptor protein may not be labelled with the acceptor, or the orientation between the donor and the acceptor may be wrong. Orientation is usually considered random, and taken into account by the κ2 factor. If the labelling is complete, as it can be expected if the cell is expressing fusion proteins of the GFP variants, the decay components represent the fractions of interacting and non-interacting donor molecules. Corrected by κ2, the amplitudes, a and b, represent the fractions of interacting and non-interacting protein molecules. The composition of the donor decay function is illustrated in the figure below.
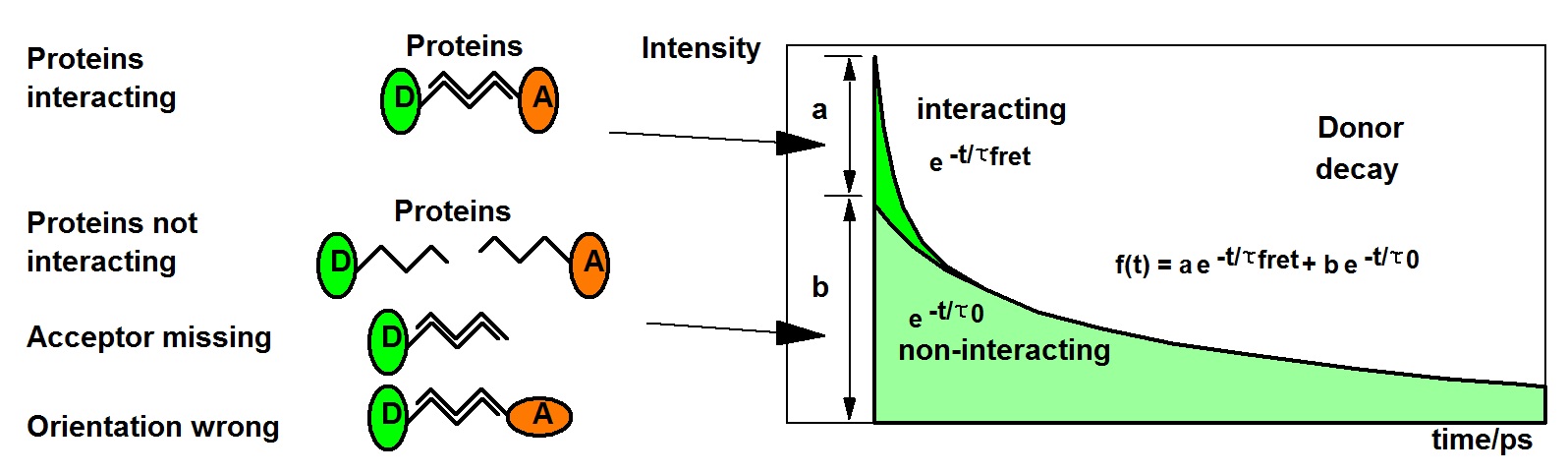
Precision measurement of the decay curves and double-exponential FLIM analysis by SPCImage NG directly deliver the classic FRET Efficiency, the FRET Efficiency of the interacting donor fraction, the donor-acceptor distance, and the relative amounts of interacting and non-interacting donor. The technique also has another charm: The lifetime of the non-interacting donor fraction is the reference lifetime for the FRET analysis. This makes the technique calibration-free. This is a significant advantage over classic FRET measurement with single-exponential decay analysis.

For more information and references please see bh TCSPC Handbook, chapter ‘Förster Resonance Energy Transfer (FRET)’.
References
References for FRET Imaging
For more references on FLIM-FRET please see bh TCSPC Handbook, chapter ‘Förster Resonance Energy Transfer (FRET)’.
- Ahmed, R. George, C.-C. Lin, K. M. Suen, J. A. Levitt, K. Suhling, J. E. Ladbury, Direct binding of Grb2 SH3 domain to FGFR2 regulates SHP2 function. Cellular Signalling 22 23-33 (2010)
- Ahmed, Z. Timsah, K. M. Suen, N. P. Cook, G. R. Lee, Chi-Chuan Lin, Mihai Gagea, A. A. Marti, J.n E. Ladbury, Grb2 monomer–dimer equilibrium determines normal versus oncogenic function. Nature Communications 6, 7354-1 to -9
- R. Alam, H. Wallrabe, Z. Svindrych, A. K. Chaudhary, K. G. Christopher, D. Chandra, A. Periasamy, Investigation of Mitochondrial Metabolic Response to Doxorubicin in Prostate Cancer Cells: An NADH, FAD and Tryptophan FLIM Assay. Scientific Reports 7 (2017)
- Amari, E. Boutant, C. Hofmann, C. Schmitt-Keichinger, L. Fernandez-Calvino, P. Didier, A. Lerich, J. Mutterer, C. L. Thomas, M. Heinlein, Y. Mely, A. J. Maule, C. Ritzenthanler, A family of plasmodesmal proteins with receptor-like properties for plant viral movement proteins. PLOS Pathogens 6 e1001119-1 to -10 (2010)
- Aow, K. Dore, R. Malinow, Conformational signaling required for synaptic plasticity by the NMDA receptor complex. PNAS Early Edition, www.pnas.org/cgi/doi/10.1073/pnas.1520029112
- J. Bacskai, J. Skoch, G.A. Hickey, R. Allen, B.T. Hyman, Fluorescence resonance energy transfer determinations using multiphoton fluorescence lifetime imaging microscopy to characterize amyloid-beta plaques, J. Biomed. Opt 8, 368-375 (2003)
- J. Bacskai, J. Skoch, G.A. Hickey, O. Berezovska, B.T. Hyman, Multiphoton imaging in mouse models of Alzheimer’s disease, Proc. SPIE, 5323, 71-76 (2004)
- Becker, K. Benndorf, A. Bergmann, C. Biskup, K. König, U. Tirlapur, T. Zimmer, FRET measurements by TCSPC laser scanning microscopy, Proc. SPIE 4431, 94-98 (2001)
- Becker, A. Bergmann, M.A. Hink, K. König, K. Benndorf, C. Biskup, Fluorescence lifetime imaging by time-correlated single photon counting, Micr. Res. Techn. 63, 58-66 (2004)
- Berezovska, P. Ramdya, J. Skoch, M.S. Wolfe, B.J. Bacskai, B.T. Hyman, Amyloid precursor protein associates with a nicastrin-dependent docking site on the presenilin 1-g-secretase complex in cells demonstrated by fluorescence lifetime imaging, J. Neurosci. 23, 4560-4566 (2003)
- Berezovska, B.J. Bacskai, B.T. Hyman, Monitoring Proteins in Intact Cells, Science of Aging Knowledge Environment, SAGE KE 14 (2003)
- Berezovska, A. Lleo, L.D. Herl, M.P. Frosch, E.A. Stern, B.J. Bacskai, B.T. Hyman, Familial Alzheimer’s disease presenilin 1 mutations cause alterations in the conformation of prenesilin and interactions with amyloid precursor protein, J. Neurosci. 25, 3009-3017 (2005)
- Biskup, L. Kelbauskas, T. Zimmer, K. Benndorf, A. Bergmann, W. Becker, J.P. Ruppersberg, C. Stockklausner, N. Klöcker, Interaction of PSD-95 with potassium channels visualized by fluorescence lifetime-based resonance energy transfer imaging, J. Biomed. Opt. 9, 735-759 (2004)
- Biskup, T. Zimmer, L. Kelbauskas, B. Hoffmann, N. Klöcker, W. Becker, A. Bergmann, K. Benndorf, Multi-Dimensional Fluorescence Lifetime and FRET Measurements. Micr. Res. Tech. 70, 403-409 (2007)
- Bosch, J. Castro, T. Saneyoshi, H. Matsuno, M. Sur, Y. Hayashi, Structural and Molecular Remodeling of Dendritic Spine Substructures during Long-Term Potentiation. Neuron 82, 12059-1 to -16 (2014)
- Calleja, S. Ameer-Beg, B. Vojnovic, R. Woscholski, J. Downwards, B. Larijani, Monitoring conformational changes of proteins in cells by fluorescence lifetime imaging microscopy, Biochem. J. 372, 33-40 (2003)
- R. Cao, H. Wallrabe, K. Siller, S. R. Alam, A. Periasamy, Single cell redox states analyzed by fluorescence lifetime metrics and tryptophan FRET interaction with NAD(P)H. Cytometry Part A, 96A, 110-121 (2019)
- Chen, A. Periasamy, Characterization of two-photon excitation fluorescence lifetime imaging microscopy for protein localization, Microsc. Res. Tech. 63, 72-80 (2004)
- Cheung, M. Gill, A. Esposito, C. F. Kaminski, N. Courousse, S. Chwetzoff, G. Trugnan, N. Keshavan, A. Lever, U. Desselberger, Rotaviruses Associate with Cellular Lipid Droplet Components To Replicate in Viroplasms, and Compounds Disrupting or Blocking Lipid Droplets Inhibit Viroplasm Formation and Viral Replication. J. Virol. 84, 6782-6798 (2010)
- N. Day, C.F. Booker, A. Periasamy, Characterization of an improved donor fluorescent protein for Förster resonance energy transfer microscopy. J. Biomed. Opt. 13(3), 031203-1 to -9 (2008)
- N. Day, F. Schaufele, Fluorescent protein tools for studying protein dynamics in living cells: A review. J. Biomed. Opt. 13(3), 031202-1 to -6 (2008)
- Dore, S. Labrecque, C. Tardif, P. De Koninck, FRET-FLIM Investigation of PSD95-NMDA Receptor Interaction in Dendritic Spines; Control by Calpain, CaMKII and Src Family Kinase. PLOS ONE 9(11), e112170 (2014)
- Dore, J. Aow, R. Malinow, Agonist binding to the NMDA receptor drives movement of its cytoplasmic domain without ion flow. PNAS Early Edition, www.pnas.org/cgi/doi/10.1073/pnas.1520023112
- R. Duncan, A. Bergmann, M.A. Cousin, D.K. Apps, M.J. Shipston, Multi-dimensional time-correlated single-photon counting (TCSPC) fluorescence lifetime imaging microscopy (FLIM) to detect FRET in cells, J. Microsc. 215, 1-12 (2004)
- D. Ellis , D. Llères, M. Denegri , A.I. Lamond , J.F. Cáceres, Spatial mapping of splicing factor complexes involved in exon and intron definition. J. Cell Biol. 181, 921-934 (2008)
- Förster, Energy migration and fluorescence. Translated by Klaus Suhling. J. Biomed. Opt. 17 011002-1 to -10 (2012)
- Fogel, S. Frere, O. Segev, S. Bharill, I. Shapira, N. Gazit, T. O’Malley, E. Slomowitz, Y. Berdichevsky, D.M. Walsh, E.Y. Isacoff, J.A. Hirsch, I. Slutsky, APP homodimers transduce an amyloid-β-mediated increase in release probability at excitatory synapses. Cell Rep. 7 1560-1576 (2014)
- H. Fox, T. Connor, M. Stiles, J. Kama, Z. Lu, K. Dorsey, G. Lieberman, E. Sapp, R.A. Cherny, M. Banks, I. Volitakis, M. DiFiglia, O. Berezovska, A.I. Bush, S.M. Hersch, Cysteine Oxidation within N-terminal Mutant Huntingtin Promotes Oligomerization and Delays Clearance of Soluble Protein. J. Biol. Chem. 286, 18320-18330 (2011)
- O. Fruhwirth, S. Ameer-Beg, R. Cook, T. Watson, T. Ng, F. Festy, Fluorescence lifetime endoscopy using TCSPC for the measurement of FRET in live cells. Opt. Expr. 18, 11148-11158 (2010)
- Ghukasyan, Y.-Y. Hsu, S.-H. Kung, F.-J. Kao, Application of fluorescence resonance energy transfer resolved by fluorescence lifetime imaging microscopy for the detection of enterovirus 71 infection in cells, J. Biomed. Opt. 12, 024016-1 to -8 (2007)
- Guardia-Laguarta, M. Coma, M. Pera, J. Clarimon, L. Sereno, J.M. Agullo, L. Molina-Porcel, E. Gallordo, A. Deng, O. Berezovska, B. Hyman, R. Blesa, T. Gomez-Isla, A. Lleo, Mild cholesterol depletion reduces amyloid-b production by impairing APP trafficking to the cell surface. J. Neurochem. 110, 220-230 (2009)
- Herl, A. V. Thomas, C. M. Lill, M. Banks, A. Deng, P. B. Jones, R. Spoelgen, B.y T. Hyman, O. Berezovska, Mutations in amyloid precursor protein affect its interactions with presenilin/g-secretase. Molecular and Cellular Neuroscience 41, 166–174 (2009)
- Ilien, N. Glasser, J.-P. Clamme, P. Didier, E. Piemont, R. Chinnappan, S. B. Daval, J.-L. Galzi, Y. Mely, Pirenzepine Promotes the Dimerization of Muscarinic M1 Receptors through a Three-step Binding Process. J. Biol. Chem. 284(29) 19533–19543, (2009)
- B. Jones, K.W. Adams, A. Rozkalne, T.L. Spires-Jones, T.T. Hshieh, T. Hashimoto1, C.A. F. von Armin, M. Mielke, B.J. Bacskai, B.T. Hyman, Apolipoprotein E: Isoform Specific Differences in Tertiary Structure and Interaction with Amyloid-b in Human Alzheimer Brain. PLoS One 6, e14586 (2011)
- Jyothikumar, Y. Sun, A. Periasamy, Investigation of tyrptopahn-NADH in live human cells using 3-photon fluorescence lifetime imaging. J. Biomed. Opt. 18(6), 060501 (2013)
- Kwaaitaal, N.F. Keinath, Simone Pajonk, C. Biskup, R. Panstruga, Combined Bimolecular Fluorescence Complementation and Förster Resonance Energy Transfer Reveals Ternary SNARE Complex Formation in Living Plant Cells. Plant Physiology 152, 1135-1147 (2010)
- V. Koushik, H. Chen, C. Thaler, H. L. Puhl, and S. S. Vogel, Cerulean, Venus, and VenusY67C FRET Reference Standards. Biophys J.: Biophysical Letters L99-L101 (2006)
- Lleo, O. Berezovska, L. Herl, S. Raju, A. Deng, B.J. Bacskai, M.P. Frosch, M. Irizarry, B.T. Hyman, Nonsteroidal anti-inflammatory drugs lower Ab42 and change presenilin 1 conformation, Nature Medicine 10, 1065-1066 (2004)
- Lleres, S. Swift, A. I. Lamond, Detecting Protein-Protein Interactions In Vivo with FRET using Multiphoton Fluorescence Lifetime Imaging Microscopy (FLIM). Curr. Protoc. Cytom. 42, 12.10.1-12.10.19 (2007)
- Lleres, J. James, S. Swift, D.G. Norman, A. I. Lamond, Quantitative analysis of chromatin compaction in living cells using FLIM-FRET. J. Cell Biol. 187, 481-191 (2009)
- Lleres, A. P. Bailly A. Perrin, D. G. Norman, D. P. Xirodimas, R. Feil, Quantitative FLIM-FRET Microscopy to Monitor Nanoscale Chromatin Compaction In Vivo Reveals Structural Roles of Condensin Complexes. Cell Reports 18, 1791-1803 (2017)
- N. Medine, A. McDonald, A, Bergmann, R. Duncan, Time-correlated single photon counting FLIM: Some considerations for Physiologists. Micr. Res. Tech. 70, 421-425 (2007)
- L. Medintz, T. Pons, K. Susumu, K. Boeneman, A.M. Dennis, D. Farrel, J.R.Deschamps, J.S. Melinger, G. Bao, H. Mattoussi, Resonance energy trensfer between luminescent quantum dots and diverse fluorescent protein acceptors. J. Phy. Chem. C 113, 18552-18560 (2009)
- Munsie, N. Caron, R. S. Atwal, I. Marsden, E. J. Wild, J. R. Bamburg, S. J. Tabrizi, R. Truant, Mutant huntingtin causes defective actin remodeling during stress: defining a new role for transglutaminase 2 in neurodegenerative disease. Human Molecular Genetics, 2011, Vol. 20, No. 10 1937–1951
- Murakoshi, S-J. Lee, R. Yasuda, Highly sensitive and quantitative FRET–FLIM imaging in single dendritic spines using improved non-radiative YFP. Brain Cell Biol. 36, 31-42 (2008)
- Murakoshi, A.C.E. Shibata, ShadowY: a dark yellow fluorescent protein for FLIM-based FRET measurement. Scientific Reports 7, 6791-1 to 10 (2017)
- 888. T. A. Nguyen, H. L. Puhl, K. Hines, D. J. Liput, S. S. Vogel, Binary-FRET reveals transient excited-state structure associated with activity-dependent CaMKII – NR2B binding and adaptation. Nature Communications 13, 6335-1 to -14 (2022)
- Pelet, M.J.R. Previte, P.T.C. So, Comparing the quantification of Förster resonance energy transfer measurement accuracies based on intensity, spectral, and lifetime imaging. J. Biomed. Opt. 11 034017-1 to -11 (2006)
- Periasamy, R.M. Clegg, eds., FLIM Microscopy in Biology and Medicine. CRC Press 2009
- Periasamy, N. Mazumder, Y. Sun, K. G. Christopher, R. N. Day, FRET Microscopy: Basics, Issues and Advantages of FLIM-FRET Imaging. In: W. Becker (ed.) Advanced time-correlated single photon counting applications. Springer, Berlin, Heidelberg, New York (2015)
- Peter, S.M. Ameer-Beg, Imaging molecular interactions by multiphoton FLIM, Biology of the Cell 96, 231-236 (2004)
- Peter, S.M. Ameer-Beg, M.K.Y. Hughes, M.D. Keppler, S. Prag, M. Marsh, B. Vojnovic, T. Ng, Multiphoton-FLIM quantifcation of the EGFP-mRFP1 FRET pair for localization of membrane receptor-kinase interactions, Biophys. J. 88, 1224-1237 (2005)
- Richert, P. Didier, H. de Rocquigny, Y. Mély, Monitoring HIV-1 Protein Oligomerization by FLIM FRET Microscopy. In: W. Becker (ed.) Advanced time-correlated single photon counting applications. Springer, Berlin, Heidelberg, New York (2015)
- Rickman, C.N. Medine, A. Bergmann, R.R. Duncan, Functionally and spatially distinct modes of MUNC18-syntaxin 1 intercation. JBC 282, 12097-12103 (2007)
- Rickman, C. N. Medine, A. R. Dun, D. J. Moulton, O. Mandula, N. D. Halemani, S. O. Rizzoli, L. H. Chamberlain, and R. R. Duncan, t-SNARE Protein Conformations Patterned by the Lipid Microenvironment. J. Biol. Chem. 285, 13535-13541 (2010)
- Rickman, R. R. Duncan, Munc18/Syntaxin Interaction Kinetics Control Secretory Vesicle Dynamics. J. Biol. Chem. 285, 3965-3972 (2010)
- L. Rusanov, A.P. Savitsky, Fluorescence resonance energy transfer between fluorescent proteins as powerful toolkits for in vivo studies. Laser Phys. Lett. 8(2) 91-102 (2011)
- Snippe, J.W. Borst, R. Goldbach, R. Kormelik, The use of fluorescence microscopy to visualise homotypic interactions of tomato spotted wilt virus nucleocapsid protein in living cells, J. Vir. Meth. 125, 12-15 (2005)
- Ueda, S. Kwok, Y. Hayashi, Application of FRET probes in the analysis of neuronal plasticity, Frontiers in neural circuits 7, 163 (2013)
- Valkonen, E. R. Kalkman, M. Saloheimo, M. Penttilä, N. D. Read, R. R. Duncan, Spatially segregated snare protein interactions in living fungal cells. JBC 282, 22775-22785 (2007)
- S. Vogel, C. Thaler, S. V. Koushik, Fanciful FRET. Sci. STKE 2006, re2 (2006)