
Principles
Table of Contents:
- Measurement of Molecular-Environment Parameters:
- pH Imaging
- Calcium Imaging
- Chloride Imaging
- Local Viscosity in Molecular Imaging
- Protein Interaction in Molecular Imaging
- Membrane Potential
Measurement of Molecular-Environment Parameters
The fluorescence lifetime of most fluorophores more or less depends on the molecular environment. FLIM can be therefore used to measure local environment parameters, such as pH, oxygen concentration, concentration of physiologically important ions, binding to RNA, DNA, proteins or lipids, local viscosity, and local refractive index. Compared with intensity-based methods fluorescence-lifetime detection has the advantage that the result gets de-coupled from the concentration of the sensor, possible absorption in the sample, intensity of the laser, focusing, detector gain and other instrumental effects. A few examples are described below.
pH Imaging
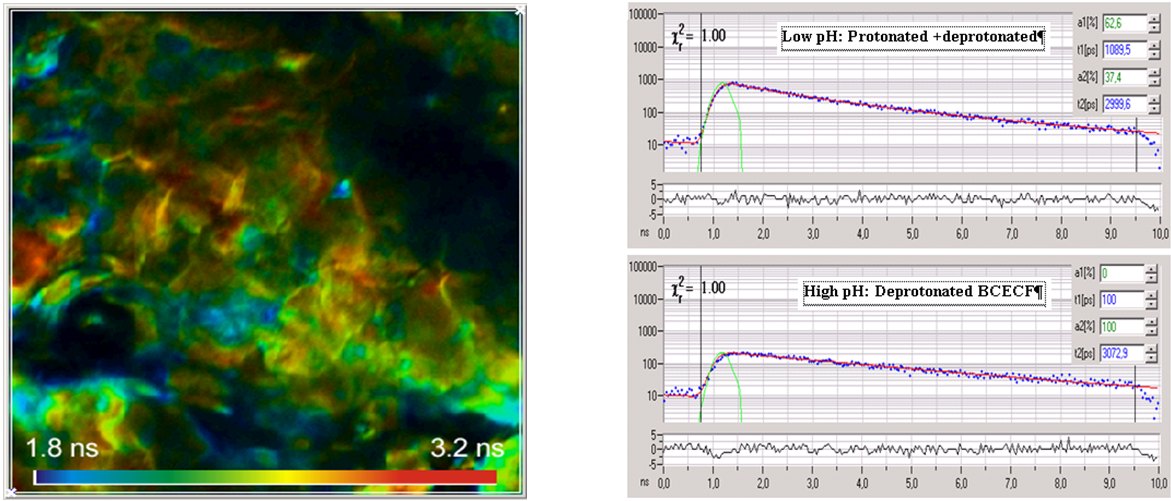
Microscopic pH imaging by FLIM can be achieved by staining the sample with a pH-sensitive fluorescent probe. These probes usually have a protonated and a deprotonated form. There is an equilibrium between both forms that depends on the pH of the local environment. If both forms have different fluorescence lifetimes the average lifetime is a direct indicator of the pH. A typical representative of the pH-sensitive dyes is 2′,7′-bis-(2-carboxyethyl)-5-(and-6)-carboxyfluorescein (BCECF). An example of pH imaging of skin tissue is shown in the figure below. A single-exponential lifetime image is shown left, decay curves from different areas of the image are shown right.
Calcium Imaging
Ca2+ ions are involved in a large number of cell functions, such as intracellular transport, membrane potential, muscle contraction, gene expression, and cell differentiation. The mechanism of the Ca2+-dependent lifetime of Ca2+ sensors is that the fluorophore has a Ca-bound and an Ca-unbound form of different fluorescence quantum efficiency and thus different fluorescence lifetimes. The fluorescence lifetime of the bound form is higher than that of the unbound form. Consequently, the net fluorescence lifetime depends on the Ca2+ concentration. The traditional Ca2+ dyes, such as Calcium Green and Oregon Green Bapta, display large lifetime changes and work beautifully for lifetime-based Ca2+ measurement. Examples are shown in the figures below
The Ca2+ concentration in cells can change within remarkably short periods of time. The Ca2+ response of live neurons to stimulation occurs within milliseconds. Nevertheless, the effects can be measured by special TCSPC FLIM techniques, such as FLITS and Temporal Mosaic FLIM. An example is shown in the figure below.

Chloride Imaging
The chloride concentration is important to the function of the neuronal system. MQAE (a quinin derivate) is used as a fluorescent probe. MQAE is quenched by Cl–, and the concentration can be calculated from the lifetime change via the Stern-Volmer relation. The figure below shows a spinal ganglion of a mouse stained with MQAE. Short lifetimes indicate high Cl– concentration and vice versa.
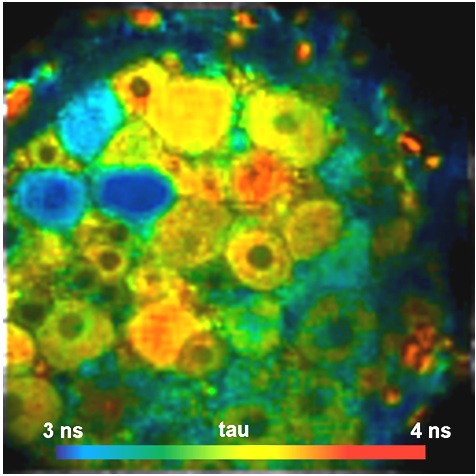
Local Viscosity in Molecular Imaging
Local viscosity has a large influence on the rates of biochemical reactions in a cell. Changes in viscosity on the sub-cellular level have been shown to accompany a wide range of cell mal-functions. Changes in viscosity have been found for hematoligic disorders, diabetes, Alz-heimer disease, liver malfunction and cancer. Viscosity on the molecular scale can be determined by ‘molecular rotors’. These are fluoro-phores which have two more or less rigid parts connected by a single bond. The two parts can rotate one against the other. Rotation is an additional relaxation pathway which competes with radiative relaxation. Ability of internal rotation therefore shortens the fluorescence lifetime. When the rotation is hindered by high viscosity of the molecular environment this relaxation pathway becomes less efficient, and the fluorescence lifetime increases. An example of a vis-cosity measurement by a Bodipy-based sensor is shown in the figure below.
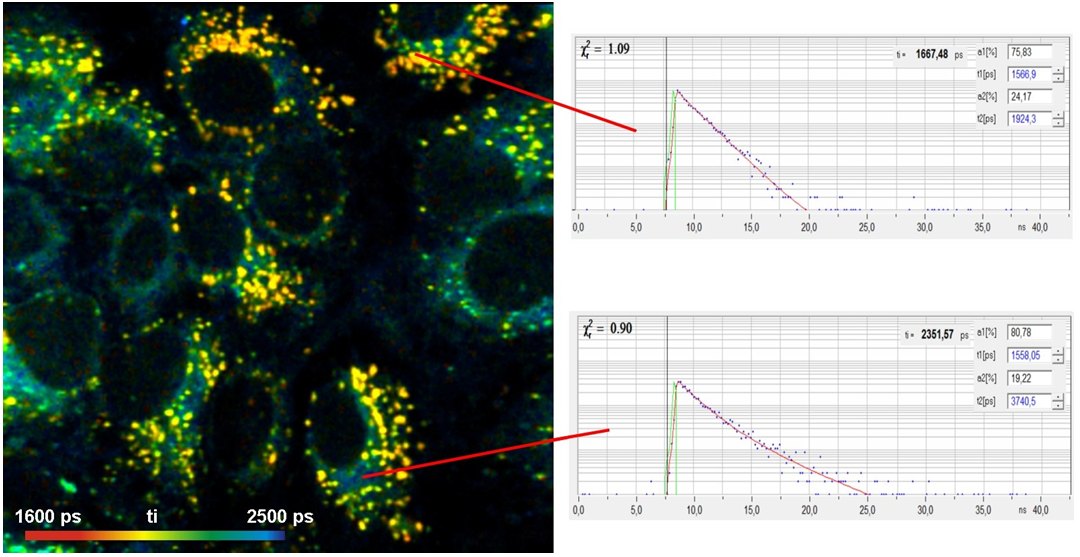
Protein Interaction in Molecular Imaging
Protein interaction is investigated by using Foerster-Resonance Energy Transfer (FRET) between a donor molecule and an acceptor molecule. The donor molelcule is attached to one protein, the acceptor molecule to another. If the proteins are connected (interacting) energy is transferred from the donor to the acceptor. The causes a decrease in the fluorescence lifetime of the donor. FRET experiments by FLIM are more quantitative than by staedy-state imaging because FLIM is independent of the concentration. Moreover, FLIM delivers not only the classic FRET efficiency but also the fraction of interacting proteins and the FRET efficiency of the interacting donor alone. FRET distances from this FRET efficiency are not offset by variable fraction of interacting proteins. Please see Protein-Interaction Experiments by Förster Resonance Energy Transfer (FRET).
Membrane Potential
Cells have concentration gradients of ions across their membranes. These gradients produce a voltage over the membrane which is characteristic of a number of fundamental cellular processes. Conventional techniques for precise measurement of membrane potentials are rare. Patch-clamp techniques are highly invasive and do not deliver spatially resolved data. Optical methods based on the fluorescence intensity exist but are not quantitative. Ratiometric intensity imaging has not yet resulted in quantitative results. A promising solution is FLIM with a voltage-sensitive dye. Therefore, FLIM is the method of choice. It is intrinsically ratiometric and thus able to deliver quantitative results. The figure below shows a FLIM image of HEK cells loaded with VoltageFluor VF2.1.Cl.

Literature:
W. Becker, The bh TCSPC Handbook
W. Becker (ed.), Advanced Time-Correlated Single-Photon Counting Applications
References
Selected References Related to Molecular Imaging by FLIM
For more references please see W. Becker, The bh TCSPC Handbook, 9ed. (2021).
- Arosio, G. Garau, F. Ricci, L. Marchetti, R. Bizzarri, R. Nifosi, F. Beltram, Spectroscopic and Structural Study of Proton and Halide Ion Cooperative Binding to GFP. Biophys. J. 93, 232-244 (2007)
- Becker, V. Shcheslavskiy, Fluorescence Lifetime Imaging with Near-Infrared Dyes. Photonics and Lasers in Medicine 4, 73-83 (2015)
- Becker, V. Shcheslavkiy, S. Frere, I. Slutsky, Spatially Resolved Recording of Transient Fluorescence-Lifetime Effects by Line-Scanning TCSPC. Microsc. Res. Techn. 77, 216-224 (2014)
- Becker, Fluorescence Lifetime Imaging – Techniques and Applications. J. Microsc. 247 (2) (2012)
- Y. Berezin, S. Achilefu, Fluorescence lifetime maesurement and biological imaging. Chem. Rev. 110(5), 2641-2684 (2010)
- Biskup, Th. Gensch, Fluorescence lifetime imaging of ions in biological tissue. In: L. Marcu. P.M.W. French, D.S. Elson, (eds.), Fluorecence lifetime spectroscopy and imaging. Principles and applications in biomedical diagnostics. CRC Press, Taylor & Francis Group, Boca Raton, London, New York (2015)
- C. Biskup, L. Kelbauskas, T. Zimmer, K. Benndorf, A. Bergmann, W. Becker, J.P. Ruppersberg, C. Stockklausner, N. Klöcker, Interaction of PSD-95 with potassium channels visualized by fluorescence lifetime-based resonance energy transfer imaging, J. Biomed. Opt. 9, 735-759 (2004)
- Douma, R.T.A. Megens, S. Reitsma, L. Prinzen, D.W. Slaaf, M.A.M.J. Van Zandvoort, Two-photon lifetime inaging of fluorescent probes in intact blood vessels: A window to sub-cellular structural information and binding status. Micr. Res. Tech. 70, 467-475 (2007)
- Frere, I. Slutsky, Calcium imaging using Transient Fluorescence-Lifetime Imaging by Line-Scanning TCSPC. In: W. Becker (ed.) Advanced time-correlated single photon counting applications. Springer, Berlin, Heidelberg, New York (2015)
- Funk, A. Woitecki, C. Franjic-Würtz, Th. Gensch, F. Möhrlein, S. Frings, Modulation of chloride homeostasis by inflammatory mediators in dorsal ganglion neurons. Molecular Pain 4:32 (2008)
- I. Garcia, P. Lanigan, M. Webb, T. G. West, J. Requejo-Isidro, E. Auksorius, C. Dunsby, M. Neil, P. French, M. A. Ferenczi, Fluorescence Lifetime Imaging to Detect Actomyosin States in Mammalian Muscle Sarcomeres. Biophys. J. 93, 2091-2101 (2007)
- Gensch, V. Untiet, A. Franzen, P. Kovermann, C. Fahlke, Determination of Intracellular Chloride Concentrations by Fluorescence Lifetime Imaging. In: W. Becker (ed.) Advanced time-correlated single photon counting applications. Springer, Berlin, Heidelberg, New York (2015)
- Gilbert, C. Franjic-Würtz, K. Funk, T. Gensch, S. Frings, F. Möhrlen, Differential maturation of chloride homeostasis in primary afferent neurons of the somatosensory system. Int. J. Devl. Neuroscience 25, 479-489 (2007)
- A. Hosny, C. Fitzgerald, A. Vysniauskas, A. Athanasiadis, T. Berkemeier, N. Uygur, U. Poeschl, M. Shiraiwa, M. Kalberer, F. D. Pope, M. K. Kuimova, Direct imaging of changes in aerosol particle viscosity upon hydration and chemical aging, Chem. Sci. 7, 1357-1367 (2016)
- A. Hosny, G. Mohamedi, P. Rademeyer, J. Owen, Y. Wu, M. X. Tang, R. J. Eckersley, E. Stride, and M. K. Kuimova, Mapping microbubble viscosity using fluorescence lifetime imaging of molecular rotors. Proc Natl Acad Sci U S A 110, 9225-9230 (2013)
- Jenkins, S. M. Borisov, D. B. Papkovsky, R. I. Dmitriev, Sulforhodamine Nanothermometer for Multiparametric Fluorescence Lifetime Imaging Microscopy. Anal. Chem. 88, 10566-10572
- V. Kuchibhotla, C.R. Lattarulo, B. Hyman, B. J. Bacskai, Synchronous hyperactivity and intercellular calcium waves in astrocytes in Alzheimer mice. Science 323, 1211-1215 (2009)
- Kuchlyan, S. Basak, D. Dutta, A. Kumar Das, D. Mal a, N. Sarkar, A new rhodamine derived fluorescent sensor: Detection of Hg2+ at cellular level. Chem. Phys. Lett. 673, 84-88 (2017)
- Kaneko, I. Putzier, S. Frings, U. B. Kaupp, Th. Gensch, Chloride Accumulation in Mammalian Olfactory Sensory Neurons, J. Neurosci., 24 7931-7938 (2004)
- Kubánková, I. López-Duarte, J. A. Bull, D. M. Vadukul, L. C. Serpell, M. de Saint Victor, E. Stride, M. K. Kuimova, Probing supramolecular protein assembly using covalently attached fluorescent molecular rotors, Biomaterials 139, 195-201 (2017)
- A. Levitt, P.-H. Chung, K. Suhling, Spectrally resolved fluorescence lifetime imaging of Nile red for measurements of intracellular polarity. J. Biomed. Opt. 20(9), 096002-1 to -11 (2015)
- Mik. A. J. Thompson, M. R. Dent, J. Hofkens and M. K. Kuimova, Measuring the viscosity of the Escherichia coli plasma membrane using molecular rotors, Biophys J 111, 1529-1540 (2016)
- Y. Chen, A. Periasamy, Characterization of two-photon excitation fluorescence lifetime imaging microscopy for protein localization, Microsc. Res. Tech. 63, 72-80 (2004)
- A. Periasamy, N. Mazumder, Y. Sun, K. G. Christopher, R. N. Day, FRET Microscopy: Basics, Issues and Advantages of FLIM-FRET Imaging. In: W. Becker (ed.) Advanced time-correlated single photon counting applications. Springer, Berlin, Heidelberg, New York (2015)
- Ponjavic, J. Dench, N. Morgan, J. S. S. Wong, In situ viscosity measurement of confined liquids. RSC Adv. 5, 99585-99593 (2015)
- Saxl, F. Khan, D.R. Matthews, Z.-L. Zhi, O. Rolinski, S. Ameer-Beg, J. Pickup, Fluorescence lifetime spectroscopy and imaging of nano-engineered glucose sensor microcapsules based on glucose/galactose-binding protein. Biosensors and Bioelectronics 24, 3229–3234 (2009)
- S. Sherin, I. López-Duarte, M. R. Dent, M. Kubánková, A. Vyšniauskas, J. A. Bull, E. S. Reshetnikova, A. S. Klymchenko, Y. P. Tsentalovich and M. K. Kuimova, Visualising membrane viscosity of porcine eye lens cells using molecular rotors, Chem. Sci. 8, 3523-3528 ( 2017)
- -F. Shen, M.-R. Tsai, S.-C. Chen, Y.-S. Leung, C.-T. Hsieh, Y.-S. Chen, F.-L. Huang, R. P. Obena, M. M. L. Zulueta, H.-Y. Huang, W.-J. Lee, K.-C. Tang, C.-T. Kung, M.-H. Chen, D.-B. Shieh, Y.-J. Chen, T.-M. Liu, P.-T. Chou, and C.-K. Sun, Imaging endogenous bilirubins with two-photon fluorescence of bilirubin dimers. Anal. Chem. 87, 7575-7582 (2015)
- Shimolina, M. A. Izquierdo, I. López-Duarte, J. Bull, M. Shirmanova, L. Klapshina, E. Zagaynova, M. K. Kuimova, Imaging tumor microscopic viscosity in vivo using molecular rotors Sci. Reports 7, 41097-1 to -5 (2017)
- M. V. Shirmanova, D. A. Gorbachev, K. S. Sarkisyan, A. P. Parnes, A. I. Gavrina, A. V. Polozova, T. F. Kovaleva, L. B. Snopova, V. V. Dudenkova, E. V. Zagaynova, K. A. Lukyanov, FUCCI‑Red: a single‑color cell cycle indicator for fluorescence lifetime imaging. Cellular and Molecular Life Sciences, https://doi.org/10.1007/s00018-020-03712-7 (2021)
- Suhling, D.M. Davis, Z. Petrasek, J. Siegel, D. Phillips, The influence of the refractive index on EGFP fluorescence lifetimes in mixtures of water and glycerol, Proc. SPIE 4259, 91-101 (2001)
- Suhling, J. Siegel, D. Phillips, P.M.W. French, S. Lévêque-Fort, S.E.D. Webb, D.M. Davis, Imaging the environment of green fluorescent protein, Biophysical J. 83, 3589-3595 (2002)
- Suhling, L. M. Hirvonen, J. A. Levitt, P.-H. Chung, C. Tregido, A. le Marois, D. Rusakov, K. Zheng, Fluorescence Lifetime Imaging (FLIM): Basic Concepts and Recent Applications. In: W. Becker (ed.) Advanced time-correlated single photon counting applications. Springer, Berlin, Heidelberg, New York (2015)
- Vyšniauskas, M. Qurashi and M. K. Kuimova, A Molecular rotor that measures dynamic changes of lipid bilayer viscosity caused by oxidative stress, Chem. Eur. J. 22, 13210–13217 (2016)
- Vyšniauskas, I. López-Duarte, N. Duchemin, T.-T. Vu, Y. Wu, E. M. Budynina, Y. A. Volkova, E. Peña Cabrera, D. E. Ramírez-Ornelas and M. K. Kuimova, Exploring viscosity, polarity and temperature sensitivity of BODIPY-based molecular rotors, Phys. Chem. Chem. Phys. (2017)
- Vyšniauskas, D. Ding, M. Qurashi, I. Boczarow, M. Balaz, H. L. Anderson; and M. K. Kuimova, Tuning the sensitivity of fluorescent porphyrin dimers to viscosity and temperature, Chem. Eur J 23, 11001 – 11010 (2017)
- A.M.J. Van Zandvoort, C.J. de Grauw, H.C. Gerritsen, J.L.V. Broers, M.G.A. Egbrink, F.C.S. Ramaekers, D.W. Slaaf, Discrimination of DNA and RNA in cells by a vital fluorescent probe: Lifetime imaging of SYTO13 in healthy and apoptotic cells, Cytometry 47, 226-232 (2002)
Products
DCS-120 MP Multiphoton FLIM System
DCS-120 Super MPC FLIM System
DCS-120 MACRO FLIM System
FLIM Systems for Zeiss LSM 710 / 780 / 880 / 980
FLIM System for Nikon A1 / C1 / C2
FLIM System for Olympus FV1000
FLIM System for Leica SP2 / SP5 / SP8
FLIM System for Nikon A1+
SPCImage NG Data Analysis Software
Molecular Imaging System
Application Notes
- Metabolic FLIM of Macroscopic Objects
- Label-Free Multiphoton FLIM of Moving Bacteria
- New SPCImage Version Combines Time-Domain Analysis with Phasor Plot
- Metabolic FLIM with Simultaneous pH Imaging
- Measurement of Membrane Potentials in Cells by TCSPC FLIM
- bh FLIM Systems Record Calcium Transients in Live Neurons